|
Updated
8/22/2012
Stabilizing
the Renewable Grid
The Off-Peak Energy Market
New wind farms are signing PPAs for energy at $50/MWh, where will
you get energy for $15/MWh?
There are numerous points throughout the discussion of WindFuels
where we note an expected price for off-peak carbon neutral energy.
In the economics discussion, the worst-case energy prices that
we project in the near term are $25/MWh, and the average case
is $15/MWh. This price expectation is our most often misunderstood
claim – and therefore the claim that is most often challenged.
Understanding the effect that deep wind power penetration has
on the electricity market is crucial to understanding one of
the most
exciting advantages of the WindFuels system – its ability
to use intermittent renewable energy whenever it is available
at a low price.
The ISO/RTO markets.
Most states within the U.S. have their electrical energy traded
through virtual markets within an Independent System Operator
(ISO) or Regional Transmission Operator (RTO). The energy is
openly bought
and sold within these virtual markets in short blocks, and the
pricing is recorded as Local Marginal Price (LMP) - the amount
a buyer would be willing to pay for one additional MWh of energy
traded within that time frame.
ISO/RTO systems are more favorable than other structures to wind
projects at large, as the larger region of established trade
allows for more transmission of energy, “smoothing” local
variability. The limit to the expansion of wind is based on its
profitability, so wind power typically continues to penetrate until
something limits its continued development. In ISO/RTO markets,
the limiter is often the final price of energy – which causes
power companies to hesitate to sign more Purchase Power Agreements
(PPA’s) for delivered energy.
When we first began investigating the economics of the WindFuels
system, we focused on the Minnesota hub in MISO (an RTO that
includes much of the industrial Northern Midwest). At the time,
Minnesota
was the state that had the greatest penetration of wind, followed
by Iowa and North Dakota. The Minnesota hub sees trades that
cover all of MN, ND, and Northern IA. This then was considered
an ideal
predictor of what an electric market with reasonably high penetration
of wind might look like if grid transmission was adequate. We’ve
continued tracking this hub. For the rest of this discussion, we
will be including data from MISO’s RT trades (wind is typically
traded in the real time or RT market) over the Minnesota hub.
More dramatic information could be shown from ERCOT data, but
in ERCOT
transmission issues dominate difficulties with wind power penetration.
There are no transmission problems for the Minnesota hub.
End-use consumers see little price difference between peak and
off-peak energy, or hour-by hour. End-use customers contract
for a given rate, and they use energy as dictated by need. A
WindFuels
system would differ because it would be available to take its
contracted energy needs at times that are solely within the discretion
of
the energy provider – who is trading over MISO. So most of
the Windfuels plant’s energy needs could be satisfied during
the cheapest hours of energy on any given day, and the energy
provider could benefit from having an easy-to-shed energy load
to help deal
with sudden price spikes.
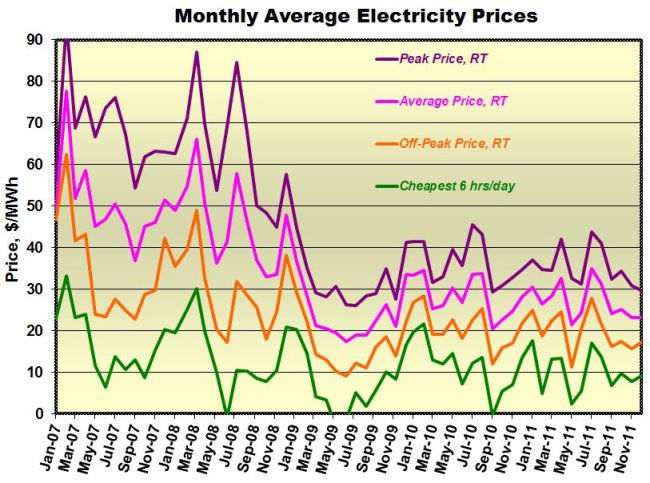
This would be a huge benefit for the power company, as it
would help draw some of the excess energy off of
the grid, forcing
the prices higher for the rest of the trades. If
the presence of an
extra few percent energy beyond demand is forcing
RT energy trades to be negatively priced for 7-40 hours/week,
then
a power company
could simply contract with a WindFuels plant for
the
excess RT energy at a very low price. On some days they'd
lose
money on
that contract, but more often they'd get far more
for that energy sold
to Windfuels then they would have gotten had they
traded it over the ISO (note the “cheapest 6 hours/day” trace in the
above graph – its average recent price is about
$8/MWh). However, there would now be a market every
day with a little
less energy traded RT, which would likely lead to
much stronger RT prices.
Thus they would generate more revenue on the improved
pricing for the rest of the RT sales than they could
ever lose
for the Windfuels
contract.
There is no better way in
an ISO for a power provider to manipulate supply at that level
without substantial
losses – in true
real time. It’s a question of game theory. If a single
power provider tried to manipulate the energy levels through
curtailing
much more of their wind electricity, that provider would lose
100% of the revenue of their curtailed wind, and the curtailment
of
other wind farms would quickly be reduced until the RT price
was back down to about the same level that it had been (price
is causing
these providers to determine when they curtail their wind). So
any provider trying to be more aggressive about wind curtailment
loses more money and advantages their competitors without getting
anything back in return. If instead the excess were simply drawn
off the grid by a controlled variable load, the wind turbines
would see less curtailment and the net prices for the rest of
the energy
traded would either be the same or higher. The power provider
could then gain both in decreased curtailment and in increased
nighttime
prices.
Negative priced energy?
You may (or may not) have heard of the concept of negative priced
energy. But there are times when power companies must literally pay others to take their energy to avoid damage to their assets
and those of their customers on the grid.
Negative pricing occurs whenever electricity providers must reduce
the net energy on the grid but do not expect to require the energy
levels to be reduced for long. Baseload power is slow to ramp up
and down, and sees a highly reduced efficiency if it is quickly
cycled.
Power companies are continually making decisions on the extent
to which their fossil power generation is tamped down versus
selling excess energy to their neighbors at prices that may not
be profitable.
As more and more wind energy is brought online in any region,
preferential treatment of local wind power will ensure that local
markets would
use some of their locally produced wind energy rather than curtailing
it, which means that more energy is now supplied in a market
that hasn’t seen any change in demand. Most grids have less than
10 minutes worth of battery storage capacity, so supply and demand
fundamentals don’t stop affecting prices at production
costs, nor do they stop influencing prices at $0/MWh.
While negative pricing is not new, intermittent wind energy has
caused the instance of negative energy to increase over the past
4 years. Figure 2 shows the price of energy at every hour during
the month of December 2011. While the resolution is limited, different
colors were used for days of the week, and different shapes were
used for different weeks of the month. The average hourly price
for the month is traced. (Note these are hourly averages, actual
prices within any given hour can swing wildly from one 5-minute
trading block to the next during a period where wind speed is inconstant).
Figure
2 shows a scatter plot of the trade LMP (local marginal price) over the Minnesota
hub in the real time market of December 2011. While
the average price of energy traded was $23.20/MWh,
it is clear from the trace that the average price for
any individual hour had a large range, and that many
hours were negatively priced.
|
Negative pricing occurs even
in regions that are seeing large curtailment rates. In fact,
throughout ERCOT, it is virtually impossible to see a day go
by without several hours of negative pricing, even though ERCOT
mandates curtailing and the ISO oversees more wind curtailment
than any other region in America.
As expected, most of the periods
of lowest-priced energy fall within periods of higher wind power
generation. As will be explained more fully in the section “Wind
Curtailment” below, the instance of negative pricing is
quite sensitive to the price of natural gas – with periods
of higher cost natural gas resulting in more negative pricing
and curtailment, and periods of lower cost natural gas resulting
in fewer cases of both of the above.
Figure 3 illustrates
the number of hours per quarter that energy has been traded at
very low prices (<$10/MWh)
and the number of hours per quarter that energy has been
traded at negative pricing.
The reduction in low and negatively priced energy seen between
2009 and 2010 was a direct result of the fact that the amount
of energy curtailed in this region nearly tripled in that time
frame.
The modest stability that occurred throughout 2011 reflected
a balance between dropping natural gas prices and increasing
wind
power penetration.

Figure
3: The red columns show the number of hours
energy prices averaged below $0/MWh throughout the
Minnesota hub, the blue columns show the number of
hours energy traded below $10/MWh.
|
As more wind power is installed, more locales will have excess
energy more often that they have to sell, increasing the supply
in the open market. This serves to increase both the instance of
negative energy prices and the amount of wind curtailment.
Wind Curtailment.
An oft-heard criticism of wind farms is that people “drive
by them every day while the wind is blowing and the turbines aren’t
spinning”. This is true, and it’s often deliberate.
The turbine blades are often pitched so that they recover less
energy or no energy from the wind, a process commonly referred
to as “wind curtailment”. The reason is obvious:
if overproduction of wind power results in negative or ultra-low
priced
energy, one way to avoid paying to get rid of excess energy is
shutting down the energy production. So curtailment happens either
to prevent or eliminate negative pricing.
When the amount of energy coming online is greater than the energy
demand, the power provider must determine whether to reduce wind-energy
generation, or ramp down fossil power (it takes 5-10 minutes to
tamp down an NG peaker, 15-20 minutes to tamp down a combined cycle
gas turbine (CCGT), and hours to tamp down a coal plant). If fossil
power is chosen to be tamped back, the price of energy will likely
stay negative as long as that process takes.
If the power company backs off too far on a coal plant in the evening,
and then the wind suddenly drops, the power company will lose money
buying emergency excess power from neighboring markets and pushing
their natural gas peaking plants to max, all while inefficiently
re-firing their coal plants. The money lost due to such a miscalculation
could easily exceed what it would cost the power company to just
keep the baseload plants burning and curtail some of the wind when
the wind blows strongly. So the power companies use the best weather
predictions available (which are very accurate for a time horizon
of a few hours,) and make the best choices they can for maximizing
profit.
The more wind power penetrates within a given regional grid,
the greater this problem becomes, and the greater the instance
of curtailment.
Clearly, if natural gas is used as the “balance power”,
one of the key economic factors becomes the price of natural gas
itself. When natural gas prices are low, then the economic penalty
of shifting more power from baseload to natural gas is reduced.
Baseload is reduced to a greater extent when higher winds are forecast – leading
to less curtailment. When NG prices are high, then there is a
greater economic penalty for using the balance power, and there
would be
more instances where the power company will curtail the wind
power instead of reducing baseload power.
This is very complex, but in order to offer a visual illustration
of these impacts we’ll look specifically at Iowa – a
plains state with no hydropower capacity that sits at the convergence
of the three largest RTO’s in the U.S.: MISO, PJM, and SPP;
so there’s no question of transmission capacity here.
The following graph shows the total amount of wind energy curtailed
in Iowa in GWh (red columns), the percentage of Iowa’s
generation that was derived from wind (green area), and the price
of natural
gas in $/MMBTU (black line).

Note that even at NG prices of below $2.00/MMBTU
Iowa is still curtailing more than 10 GWh/month. When gas prices
were $4/MMBTU,
there was 169 GWh of curtailed wind energy because the wind power
penetration increased from 14.4% to 17.4%. Currently ~25% of
the state’s energy is derived from wind. An increase in
natural gas prices should result in far higher curtailment then
was seen previously at $4.00/MMBTU, as the wind power percentage
of total generation has increased dramatically.
WindFuels would end curtailments.
We expect that a major power provider would have a strong incentive
to contract with a WindFuels plant. In cases where excess wind
production begins to threaten grid stability, rather than curtailing
the wind they could merely direct excess energy to the electrolyzers
at a nearby WindFuels facility. This energy would be contracted
for a very low price, as the alternative would be curtailment – which
has zero value – or actually see the market price go negative.
In return, the power company could get SOME return on those hours
of wind power rather than none or negative, and they would also
be eligible to receive credit for renewable energy generation being
delivered to the grid – whether that would serve to satisfy
mandates or offer subsidies or tax credits.
There is a beautiful synergy here. If natural gas is cheap, a CARMA
plant would derive most of its feedstock from natural gas, while
purchasing some grid energy when the power company would otherwise
curtail their winds. If natural gas starts increasing in price,
the amount of extremely low cost grid energy will increase non-linearly
and the CARMA plant can use more cheap grid energy and less NG.
The other advantage for the power company is that there is now
the potential for a more stable grid at large, with the power company
having the ability to adjust the demand from electrolyzers from
2% to 100% or anywhere in between with <100 millisecond (ms)
warning. This would enable them to efficiently plan for operations
and run all of their baseload thermal systems at maximum efficiency
and effectiveness, while smoothing the way for much greater wind
penetration at maintained stability.
There is clearly a strong incentive for power providers to offer
very low energy pricing for WindFuels whenever RT prices are very
low, and there is enough curtailed wind energy NOW (even with very
low-priced natural gas) to provide for the needs of scores of WindFuels
plants throughout the Midwest.
Climate Benefit Note: if a new WindFuels plant is brought online
within a region and consumes 100 GWh from the grid with specific
timing so that there is 100 GWh less wind curtailment, then no
additional fossil energy would be used to provide that portion
of energy consumed by the WindFuels plant. The actual impact will
be greater than this, as a more stable grid requiring less curtailment
would encourage more development of wind within a grid – allowing
more fossil energy to be abated than could ever have occurred without
WindFuels plants being present.
Wasted Electrical Energy.
Keep in mind that during these times of high winds and excess power,
power companies are losing money hand over fist. Baseload power
costs money, and power companies have to pay the ISOs in order
to trade energy, which they are often trading at negative value.
When energy is traded over the ISOs at such times, it is often “purchased” by
neighboring municipalities, who now have excess power that they
must “sell” at a slightly higher price to their neighbors… in
effect a series of fees paid to the ISO to “push” the
energy to outlying regions that are not suffering from an energy
glut. The transmission may be through lines and transformers that
are near capacity, thereby imposing additional costs on the trading.
But other means of dealing with excess energy abound. It’s
fairly easy to imagine that every light in every facility owned
by a power company will be shining brightly before excess power
gets “sold” for a negative price. The same is undoubtedly
true with excess AC/climate control, water heating, and whatever
else can be done with the energy, including simply running large
amounts of energy through resistor banks and heating the parking
lots.
If the energy is being “sold” at negative pricing,
it’s equally easy to imagine the co-ops who “purchase” this
energy would also be motivated to use this energy even if they
don’t have sufficient demand within their customer base,
and they too would use lighting, heating, air conditioning, and
any other power draw they could find to utilize or waste this electricity
in the middle of the night.
This is why it is not uncommon to see areas that boast of “green” initiatives
end up having tremendous amounts of lights burning in every city
building through the night, or large outdoor heating vents operating
at odd times throughout the night.
There is no way to determine exactly how much electricity is wasted,
but there is a certain financial motivation, and compelling anecdotal
evidence throughout the wind corridor.
As wind power continues to build out and develop a deeper penetration
into regional grid portfolios, all three of these issues that are
currently costing power companies – curtailment, negative
energy pricing, and electricity wasting – will continue to
increase. A low-cost contract for variable power demand during
excess generation periods would be far more profitable for the
power companies in all cases. We expect there will be no difficulty
getting favorable contracts for at least the next several decades.
Won’t
they simply stop building wind farms?
The immediate reaction from most investors
after they learn about some of the difficulties facing
high wind
penetration
into the grid is: clearly this cannot last! They’ll
stop building wind power until some “fix” is
found. The DOE apparently agrees with this assessment.
However, the DOE’s record of projecting renewable
energy installations may be even worse than their record
on projecting oil prices, and they have had a history of
being irrationally bearish on wind in particular, as we’ll
show...
Even as recently as early 2008, the DOE’s “high
economic growth case” in their Annual Energy Outlook
(AEO) 2008 was projecting a total wind capacity of 27.3
GW by 2010 and 34.6 GW by 2020. According to AEO 2008 we
would see 94 TWh generated in 2019.
The projections for wind energy generation according to
the AEO 2011 are shown in Table 1. The boldfaced years
represent actual data, the remaining are projections calculated
from the 4th quarter of 2010.
The actual data from 2010 shows that the year ended with
wind generation totaling 94.6 TWh. At the end of the second
quarter of 2012, the total installed capacity across America
was 50 GW, a milestone that the DOE stated just 16 months
earlier would not be reached for another 11 years.
While the prediction of a complete cessation of any new
installations in wind power may seem shocking, the DOE
has been predicting just such an event for years. Each
year with the new release of the AEO, they merely advance
the year in which all wind growth stops. The DOE’s
2012 AEO has remained true to form, projecting 53 GW of
installed wind power by the end of 2012, and 54 GW of installed
wind power by the end of 2020. There’s currently
another 10 GW of wind power under construction in the U.S.,
which will certainly be completed within the next 16 months.
It seems obvious that this kind of projection is more a
reflection of bias at the DOE rather than a serious attempt
to project the growth of wind. This may seem to be picking
on the DOE excessively, but it is important to realize
that they have historically been further afield in their
projections for renewable energy than any other analysts,
including coal and natural gas lobbies.
|
Year |
Average
Installed Capacity |
Total
Generation |
2008 |
24.89 |
55.42 |
2009 |
31.45 |
70.82 |
2010 |
37.49 |
91.25 |
2011 |
41.62 |
109.31 |
2012 |
48.9 |
141.78 |
2013 |
48.9 |
141.77 |
2014 |
48.9 |
141.77 |
2015 |
48.9 |
141.77 |
2016 |
48.9 |
141.77 |
2017 |
48.9 |
141.78 |
2018 |
48.9 |
141.78 |
2019 |
48.9 |
141.78 |
2020 |
49.01 |
142.16 |
2021 |
49.01 |
142.16 |
2022 |
49.64 |
144.32 |
2023 |
50.3 |
146.6 |
2024 |
50.78 |
148.46 |
2025 |
51.56 |
150.73 |
Table
1: Unreasonably pessimistic projections
from the DOE AEO 2011
for wind development in the U.S.
|
You
still haven’t explained why they won’t just stop
building wind farms.
It boils down to government intervention. Thirty-eight states
have renewable portfolio standards (RPSs, sometimes called
RESs, renewable electricity standards). For these states, the
power companies are mandated to utilize more renewable energy,
whether there is a cost justification in doing so or not. This
has been the dominant driving force behind both the wind and
solar industries for the past decade, and more states are either
adopting or expanding RPSs every year. After the record-shattering
heat wave/drought of 2012, it’s likely that resistance
to measures reducing carbon emissions should decrease throughout
the wind belt, and even more aggressive RPS legislation may
come into play.
Table 2 was compiled (mid-2012) to help give a better appreciation
for how much renewable energy must be brought online in the
coming years – in order to comply with government mandates.
Some RPSs specify installed capacity rather than percentage
of energy produced. Many specify minimum portions for certain
industries, and in some cases a date is specified so that only
newer installations can count towards this mandate.
State |
|
|
Specified non-wind |
Unspecified renewable energy needed
to fulfill RPS assuming 0.8% annual growth |
Mandated year of compliance |
Amount
of new renewable energy needed/year |
|
(GWh) |
% |
% |
(GWh) |
|
(GWh) |
AZ |
74,612 |
15.0% |
4.5% |
8,234 |
2025 |
588.2 |
CA |
251,336 |
33.0% |
|
16,238 |
2020 |
1,804.2 |
CO |
53,299 |
30.0% |
3.0% |
8,359 |
2020 |
928.7 |
CT |
29,911 |
27.0% |
7.0% |
5,655 |
2020 |
628.4 |
DC |
11,562 |
20.0% |
2.5% |
2,169 |
2020 |
241.0 |
DE |
11,522 |
25.0% |
3.5% |
2,610 |
2025 |
186.4 |
HI |
9,961 |
40.0% |
|
3,689 |
2030 |
194.1 |
IA |
56,938 |
105 MW |
|
Satisfied |
Satisfied |
|
IL |
141,954 |
25.0% |
7.0% |
21,293 |
2025 |
1,521.0 |
IN |
104,721 |
10.0% |
|
5,113 |
2025 |
365.2 |
KS |
45,565 |
20% Peak Capacity |
|
|
|
|
MA |
55,070 |
22.1% |
7.1% |
7,528 |
2020 |
836.5 |
MD |
65,581 |
22.0% |
2.0% |
13,207 |
2022 |
1,200.6 |
ME |
11,411 |
40.0% |
30.0% |
483 |
2017 |
80.4 |
MI |
104,632 |
10.0% |
|
5,466 |
2015 |
1,366.4 |
MN |
67,904 |
25.0% |
|
12,051 |
2025 |
860.8 |
MO |
83,837 |
15.0% |
0.3% |
10,832 |
2021 |
1,083.2 |
MT |
13,796 |
15.0% |
|
890 |
2015 |
222.4 |
NC |
131,879 |
12.5% |
1.2% |
13,979 |
2021 |
1,397.9 |
ND |
13,710 |
10.0% |
|
Satisfied |
Satisfied |
|
NH |
10,864 |
24.8% |
9.8% |
543 |
2025 |
38.8 |
NJ |
76,759 |
24.5% |
6.6% |
13,117 |
2020 |
1,457.4 |
NM |
22,987 |
20.0% |
6.6% |
1,100 |
2020 |
122.2 |
NV |
33,887 |
25.0% |
1.5% |
5,892 |
2025 |
420.9 |
NY |
143,663 |
30.0% |
21.7% |
6,460 |
2015 |
1,615.0 |
OH |
154,111 |
12.5% |
0.5% |
19,402 |
2025 |
1,385.9 |
OK |
59,418 |
15.0% |
|
1,471 |
2015 |
367.6 |
OR |
47,131 |
25.0% |
|
7,418 |
2025 |
529.9 |
PA |
148,840 |
18.0% |
10.5% |
8,254 |
2021 |
825.4 |
RI |
7,710 |
16.0% |
|
1,166 |
2019 |
145.7 |
SD |
11,542 |
10.0% |
|
Satisfied |
2015 |
|
TX |
364,505 |
10,000 MW |
|
Satisfied |
Satisfied |
|
UT |
28,867 |
20.0% |
|
5,305 |
2025 |
378.9 |
VA |
111,580 |
15.0% |
|
14,770 |
2025 |
1,055.0 |
VT |
5,537 |
20.0% |
|
694 |
2017 |
115.6 |
WA |
93,075 |
15.0% |
|
6,533 |
2020 |
725.9 |
WI |
68,695 |
10.0% |
|
4,478 |
2015 |
1,119.6 |
WV |
31,264 |
25.0% |
2.5% |
5,212 |
2025 |
372.3 |
Total |
2,759,636 |
|
|
|
|
|
Table
2: The passed state RPS mandates and goals as of mid-2012,
and the portion of those mandates that may be satisfied
by wind. |
Because of this, a state like Washington – which
derives over 60% of its energy from renewable sources – still
is not achieving its 15% RPS mandate. In all cases, while compiling
Table 2, we were careful to only factor in the portion of RPS
mandates that could be fulfilled by wind energy.
Effort went into accounting for the amount of currently installed
renewable energy that counted towards the RPS, and energy that
did not count towards the RPS was not considered. In order
to adhere to all regulations and goals that are currently on
the books, the U.S. will have to average an additional 24.2
TWh of renewable energy/year.
Between 2000 and 2010, America saw a total electricity demand
increase of 8.4%, and that time frame oversaw a very sluggish
economic growth that both began and ended with recessions.
The fifth column in Table 2 shows what must be implemented
if demand growth in the next decade merely equals that of the
previous one.
Even based on this conservative growth assumption, just to
satisfy current RES mandates 24.2 TWh of additional renewable
energy will have to be generated from new sources EVERY YEAR – not
counting the solar, hydro, distributed generation, farm waste,
and other itemized sources within these state mandates.
A quick look at the previous two decades should help us understand
the potential growth of many of these renewable options. Table
3 was compiled to demonstrate the growth and/or contraction
of the most popular renewable energy technologies.
Table
3:
Electrical
energy (TWh/yr) generated from renewable resources
in the U.S. |
Year |
Hydropower |
Wind |
Solar |
Geothermal |
Total Biomass |
1993 |
280.5 |
3.0 |
0.5 |
16.8 |
56.0 |
.... |
|
|
|
|
|
1997 |
356.5 |
3.3
|
0.5 |
14.7 |
58.7 |
... |
|
|
|
|
|
2000 |
275.6 |
5.6 |
0.5 |
14.1 |
60.7 |
2002 |
264.3 |
10.4 |
0.6 |
14.5 |
53.7 |
2004 |
268.4 |
14.1 |
0.6 |
14.8 |
53.5 |
2006 |
289.2 |
26.6 |
0.5 |
14.6 |
54.9 |
2007 |
247.5 |
34.4 |
0.6 |
14.6 |
55.5 |
2008 |
254.8 |
55.4 |
0.9 |
14.8 |
55.0 |
2009 |
273.4 |
73.9 |
0.9 |
15.0 |
54.5 |
2010
|
257.1
|
94.6
|
1.3
|
15.7
|
56.5
|
2011 |
325.1 |
119.7 |
1.8 |
16.7 |
56.7 |
Change 2000-2011
|
+49.5
(+18.0%)
|
+114.1
(+2038%)
|
+1.3
(+260%)
|
+2.6
(+18.4%)
|
-4.0
(-6.6%)
|
|
|
|
|
|
|
January-May
|
Hydropower
|
Wind
|
Solar
|
Geothermal
|
Total Biomass
|
2011 |
147.3 |
53.8 |
0.6 |
7.0 |
22.7 |
2012 |
126.8 |
63.5 |
1.2 |
7.0 |
25.7 |
Hydropower in America peaked in 1997
at 356.5 TWh. Weather variation shows as much as a 68 TWh
variation in yield for any given year, but the average yield
over the last 11 years has been 267.5 TWh – nearly
90 TWh below the 1997 peak. Water demand for irrigation and
consumption have decreased the volume of water passing through
the dams, and more dams have been decommissioned and destroyed
than built in the last decade. These trends are likely to
continue in the U.S., and 2020 seems likely to see less energy
from hydropower than what was averaged over the last decade.
Solar power has only recently seen build rates that outpaced
the degradation of earlier panels, and it is still insignificant.
Most solar development in the U.S. over the next decade will
be built to comply with technology-specific line items within
state’s RES mandates, and this additional generation
will be counted towards those mandates, not the generation
requirements calculated here.
Geothermal power peaked in 1993, but there has been recent
activity suggesting that this will be a growth technology for
a while. Growth over the last year has accelerated to a rate
of 6% annual growth. If this accelerated rate continues, then
a total of 11-12 additional TWh compared to that seen in 2010
can be expected by 2020.
Biomass-sourced electricity peaked in 2000 at 60.7 TWh. Though
wood co-firing has remained consistent, other sources of biomass
have receded due to competition from biofuels. Biomass has
averaged 54 TWh/year over the past decade.
It should be noted that considering only these most often discussed
sources of renewable energy – hydropower, solar, wind,
geothermal, and biomass – more renewable electricity
was generated in 1997 than in 2010. The only renewable energy
technology that saw significant growth within the last decade
is wind power – growing at an average of 32%/yr in the
U.S for the past 11 years. Wind will certainly comprise a super-majority
of the renewable energy that is brought online to meet the
additional 24.2 TWh/yr currently mandated.
Therefore, if we were to assume that wind comprised all but
~20 TWh/year of the mandated energy generation over the next
decade, there would still need to be at least 7 GW/yr installed
across America in order to achieve compliance. More aggressive
RPS mandates may be coming as the reality of global warming
sets in.
The wind farms are coming, whether or not the power companies
have any viable integration solutions.
The
high cost of “peaker” energy. “ Peak energy” is
costly because power companies are often forced to build “peaker” natural
gas power plants to service only a few hours of the day.
This means that these new plants will only operate between
4-25% capacity factor. Rules from the North American Electric
Reliability Corporation (NERC) require that a minimum capacity
of reserve be built out to insure that summer peak demands
can be reliably met. This is essential, as it reduces the
likelihood of roaming blackouts in the summer, but it is
also costly. Invariably, the power companies will make
these plants as cheaply as possible and they (1) consistently
have high NOX emissions, (2) often exceed SO2 and other
harmful emissions of baseload plants even though they usually
are gas-burning, and (3) have low efficiencies. In some
cases, there are still diesel generators serving as peakers. |
NERC Region |
Discount
rate for wind |
MRO |
8.0% |
SPP |
8.2% |
ERCOT |
8.7% |
SERC |
9.9% |
NPCC |
13.2% |
RFC |
16.6% |
WECC |
18.5% |
Table 4: the discount rate applied
to
wind capacity for minimum
reserve capacity compliance. |
Increased
wind penetration is changing the game here as well. Power companies “discount” the
capacity of wind when it comes to calculations for minimum
compliance levels. The extent to which wind is discounted varies
per NERC region, but they all fall between 8 and 18.5%, and
they typically drop as wind energy penetrates more deeply within
the region. What this means, is that if a 100 MW wind farm
is brought online in Nebraska (part of the MRO region), then
power companies only get credit for 8 MW of new capacity in
the region, and they must keep their fossil plants on standby
to comply with minimum reserve capacity requirements. The typical
capacity factor for new wind farms (before curtailment) is
32-35%, so clearly having only an 8% credit will result in
having too much capacity most of the time.
This is eliminating the need for new peaker plants in any region
that has deep wind penetration, and as shown in Figure
5, the
effect on grid energy prices is little short of shocking. As
is the case with most of the rest of the price data, the impact
on prices was tempered by increased curtailment.
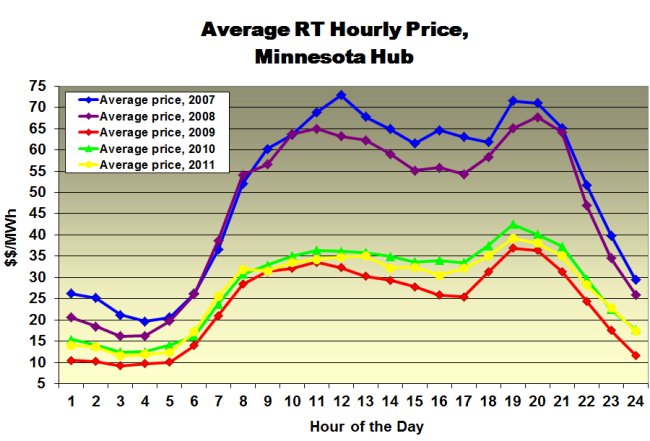
Within MISO, more than 50% of wind curtailment occurs during
peak hours. Using wind curtailment has supplanted using
rapidly ramping/tamping peaker plants to balance hour-by-hour
supply
and demand loads. In some cases it is less expensive to
over-build wind and curtail it than it is to use a
gas peaker.
The Un-viability of Conventional Energy Storage.
What increasing wind penetration has done is render
traditional energy storage completely unviable in those regions.
Referring
to Figure 5 tells part of the story; in 2007-2008, power
companies and co-ops bought and sold energy to one another
at prices averaging $60.24/MWh, during peak hours and prices
averaging $24.12/MWh during off-peak hours, a difference
of $36.12/MWh. After the market became saturated with wind,
peak-hour prices for all of 2009-2011 averaged only $32.52/MWh,
and off-peak hours averaged only $14.10/MWh, for a difference
of only $18.42/MWh. That’s a $17.70/MWh average loss
in potential revenue if the technology’s business model
is to sell energy back to the grid during the “high
priced” peak hours. Figure 6 brings this point home
even more bluntly by displaying the average of the highest
and lowest hours of each day and then tracing the average
marginal profit of a hypothetical energy storage system which
bought energy at the lowest possible price and managed with
perfect timing to sell that energy back to the grid at precisely
the highest possible price (which could not happen).
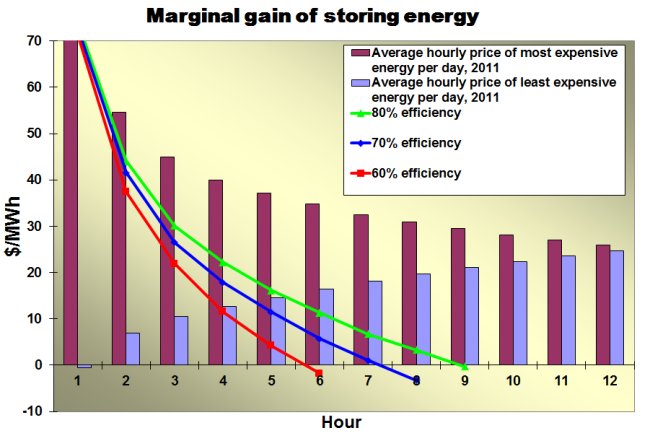
As seen from this data, purchasing grid energy and then selling
it back during peak hours (again assuming perfect timing) does
not allow much profit potential. This information should be
especially troubling for those hoping that conventional energy
storage will play a major role in integration and stability
for increased wind penetration. We have investigated the cost
of grid-level energy storage (our ASME ES2010 energy storage
paper is available here). The findings of our work have proven
to be more discerning than the traditional rhetoric that is
found elsewhere. Reviewing the LMP pricing data, a clear pattern
emerges that holds even as the overall price shifts radically
from year to year. During the warmer periods (second and third
quarters), there is a single trough in pricing, and the price
gradually increases to a single peak and then gradually descends.
During the cold periods (the first and fourth quarters of each
year), there are two peaks and two troughs. Hence, an energy
storage option that would take electrical energy from the grid
during low-price hours and sell that energy back during high-priced
hours would only cycle once per day during warm months and
twice per day during colder months. This changes the levelized
cost of energy storage considerably. For more details, the
interested reader is referred to our ASME ES2010 paper.
For WindFuels, the cost of delivered energy is similar to that
for pumped hydrostorage, but unlike all of the other options,
the stored energy will not be sold back into the local grid.
The storage for gasoline, diesel, jet fuel, and other liquid
fuels is inexpensive enough that the products can be stored
(perhaps 5-8 months) until the front-month contract is ideal
to sell. Conventional energy storage, on the other hand, must
sell the energy back into the grid, and the storage cost is
far too high to hold it for more than half a day.
Gasoline sold for ~$3.50/gallon during 2011, which works out
to ~$100/MWh. While we fully expect the price of oil and petro-products
to increase steadily over the next 5 years, the price is currently
sufficiently high to illustrate the advantage of liquid fuels
energy storage. (We expect that by 2015 the market price for
transportation fuels will be at least $150/MWh.)
Conclusions.
We have shown that, absent Windfuels, mandated RES policies
will lead to increased curtailment, increased availability
of negative-priced electricity, and reduced opportunities for
conventional energy-storage technologies to operate profitably.
These trends are expected to continue for at least several
decades, and these trends, along with the steadily increasing
price of liquid transportation fuels, establish an undeniably
strong economic and climate-benefit argument for synthesizing
standard fuels from clean, off-peak wind energy and CO2.
References:
X Lu, MB McElroy, and J Kiviluoma, “Global
potential for wind-generated electricity”, PNAS, 10.1073,
2009.
ED Delarue, PJ Luickx, and WD D’haeseleer, “The
actual effect of wind power on overall electricity generation
costs and CO2 emissions”, Energy Conv. and Mngmt 50,
1450-1456, 2009.
http://www.eia.doe.gov/ceaf/electricity/epm/table5_6_b.html
http://navigator.awstruewind.com/
MISO, https://www.midwestiso.org/Library/MarketReports/Pages/MarketReports.aspx
http://www.greentechmedia.com/articles/texas-wind-farms-bring-free-energy-and-cash-bonuses--5347.html
http://www.awea.org/faq/wwt_costs.html
http://www.thefreelibrary.com/A+novel+PSO+algorithm+for+optimal+production+cost+of+the+power...-a0216183027
http://ucsusa.org/clean_energy/solutions/big_picture_solutions/production-tax-credit-for.html
http://www.renewableenergyworld.com/rea/magazine/story?id=53498
http://dsireusa.org/
http://www.eia.doe.gov/cneaf/electricity/epa/generation_state_mon.xls
http://www.eia.doe.gov/todayinenergy/detail.cfm?id=1370
http://www.eia.doe.gov/cneaf/electricity/page/sales_revenue.xls
|
 |

|
RTO's and ISO's control
a large percentage of the electricity sold in America.
The largest RTO/ISO's include:
PJM serves
portions or all of DE, IL, IN, KY, MD, MI, NJ, NC, OH,
PA, TN, VA, WV, and DC.
MISO serves
portions or all of IA, IL, IN, MI, MN, MO, MT, NE, ND,
OH, SD, and WI.
CAISO serves
the state of California.
ERCOT serves
most of the state of Texas.
SPP serves
portions or all of AK, KS, LA, MO, NE, NM, OK, and TX
(most of the panhandle).
NYISO serves
the state of New York.
ISONE serves
all of CT, MA, ME, NH, RI, and VT.
|
|
Unlike water and gas, which are fed from huge reservoirs,
electricity must always flow. It cannot remain unused
within the power lines. Electricity must instantaneously
be used or it may damage assets connected to the grid.
Whenever generation exceeds demand the excess must be
dissipated, even at a cost.
Power companies may avoid this by curtailing wind energy,
or they may sell the energy at a significant loss.
|
|
|
|
|
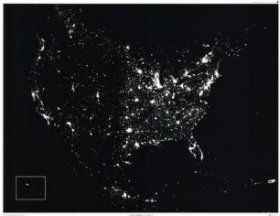
Many nights the lights in the Midwest actually are
brighter than those seen in the Northeast or West Coast
- dispite the enormous difference in population density
and wealth.
photo courtesy of NASA
|
|
|
The wind resource map shown
here - courtesy of NREL - displays the available wind resource
at 80 m. Modern wind turbines hubs are now over 100 m, so
the available resource is far greater than that shown here.
|
|
|
|
|
Hydropower
can be a mixed blessing for power companies attempting
to deal with wind integration. Weather variation can
be quite extreme. The spring of 2011 saw record high river
levels. The Bonneville Power
Administration
(BPA) made a controversial decision to respond to this
unusually high generation by breaking their PPA contracts
and shutting down the local wind farms.
(This resulted in lawsuits.)
|
|
|
|
|
|
|
|
Pumped
hydrostorage is the grid-to-grid option that has the best
overall economic merit (though it would still show a net
loss trading in ISO's with high wind penetration).
However, most of the Midwest that is having difficulty
with grid saturation is not well suited for pumped hydrostorage.
Pumped hydro needs a very significant elevation change.
|
|
|
  |